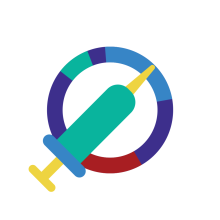
Article
Unlocking the promise of mRNA vaccines with gene synthesis technology
The COVID-19 pandemic threw a spotlight on the potential for mRNA vaccines as those developed by Pfizer/BioNTech and Moderna have been instrumental to the global vaccination programs that have prevented the deaths of millions1 and enabled the relaxation of population restrictions. However, prior to the pandemic, mRNA vaccine technology had been in development for years as scientists explored their application in a range of infectious diseases and cancers. There remains huge optimism about the potential of this technology as numerous candidates are in trials for a range of infectious diseases and cancer.
The excitement around mRNA vaccines in part comes about because of their potential to deliver significant time and efficiency savings in comparison with traditional vaccine technologies. Whereas traditional vaccine development spans an average of 10 years, COVID-19 vaccines were granted approval by regulatory bodies in the UK, US and Europe just 11 months after the SARS-CoV2 sequence became available.2 Whilst flexibility in the regulatory approach was critical to this rapid approval, the synthetic biology techniques applied to developing such a novel therapy enabled development cycles of months instead of years for traditional approaches. This speed gives mRNA vaccines some distinct advantages: not only can they be used to rapidly respond to emerging threats, but their rapid speed for development allows for their potential use in personalized therapy.
However, realizing these potential benefits requires new technology that can enable their development at much greater scale – something not possible with current technology.
Key applications will drive future mRNA vaccine growth
Vaccine manufacture is typically lengthy and expensive, and new vaccine approaches are needed to improve response times to threats and enable vaccination worldwide, particularly as the frequency of pandemic-capable diseases is increasing.3 In addition, there is a large medical unmet need with the potential to be addressed through vaccines – from infectious diseases for which there is currently no vaccine, such as malaria and HIV, through to personalized medicine for cancer. The advantages of mRNA vaccines mean that they are well suited to fill this need, and as such there is huge growth potential in mRNA vaccines across a range of applications with the antibody therapeutics market predicted to grow at 13% annually over the next decade, to be worth over $820 billion by 2033.
Before the COVID-19 pandemic hit, much research into mRNA vaccines was for their use as cancer vaccines that work by training the immune system to attack cells with cancer-specific antigens. One approach is to present antigens that are universally expressed in cancer cells but not in healthy cells; using this approach, a melanoma vaccine currently in clinical trials targets four antigens that cover more than 90% of patients.4 Alternatively, personalized vaccines can target unique mutations within a patient’s cancer. This personalized approach requires a particularly quick turnaround to allow the unique profile of the patient’s cancer to be reflected in the vaccine.
mRNA vaccines also have the potential to transform the prevention of influenza. Despite preventing tens of thousands of hospitalizations every year, the average effectiveness of these vaccines is estimated to be only 43% due to the virus rapidly evolving.5 mRNA technology has the potential to improve this significantly. Firstly, as the development time for mRNA vaccines is much shorter than traditional vaccines, scientists can select the strains to target much closer to the time when they will need to be effective. Secondly, as mRNA vaccine technology lends itself to the development of multi-valent vaccines that can target more antigens, multiple strains can targeted at once, even leading to the development of “universal” flu vaccines that are currently in trials.6,7
Scaling mRNA vaccine development requires improved gene synthesis technology
mRNA vaccine development begins with the design of a DNA template that contains the antigen sequence. Once the target is known, a DNA sequence is designed and synthesized, and the mRNA is transcribed in vitro from a template. The mRNA is then injected into the patient in the form of a vaccine and the antigen encoding sequence is transcribed so that the body mounts an immune response to the antigen that is necessary for future protection.8
While the development of mRNA vaccines is faster and more straightforward than many other types of vaccine,9 it is not without challenges. Vaccine design is a key contributor to the timeframes generally needed to deliver a new vaccine and developing a vaccine with the desired properties involves sequence optimization for stability, expression levels and efficacy.10,11 Despite the success of the COVID-19 vaccines, there is still significant room for optimization, particularly to address challenges associated with vaccine stability,12 which highlights the need for ongoing sequence optimization in mRNA vaccine templates.
Machine learning represents an exciting recent development for sequence optimization,13 but sequences ultimately need to be tested to see if the desired outcome is achieved, and this iterative design requires ready access to gene-length DNA. This represents a major bottleneck as the number of candidates that can be tested is limited by the rate that DNA can be assembled. Although the speed and scale of development of the COVID-19 vaccines was exceptional, this response was possible through existing infrastructure built prior to the emergence of SARS-CoV-2 to support iterative design.10 Biofoundries – facilities with high-throughput bioengineering and robotic capabilities – pivoted and expanded global efforts to scale vaccine manufacture.
While the need to pivot resources in this manner was obvious during the pandemic, it doesn’t provide a solution for addressing the extensive other health challenges that mRNA vaccines are poised to help. Furthermore, as mRNA vaccines are trialed as personalized cancer therapies that target tumor antigens unique to the patient, the demand for the synthesis of unique DNA templates has the potential to explode. Enabling mRNA vaccine development at scale – both for broad application and personalized medicine – requires access to gene-length DNA far beyond the levels currently available today.
Unlocking potential with new gene synthesis technology
Due to the resources and labor required, DNA synthesis and assembly have become centralized services, provided by a handful of reagent manufacturers. This holds up mRNA vaccine development pipelines as testing new sequences involves waiting for the delivery of DNA, which becomes more of a bottleneck the longer the required DNA template is (Figure 1). With DNA templates ranging from around 1kb to over 3.5kb long, this represents a significant challenge for mRNA vaccine development.
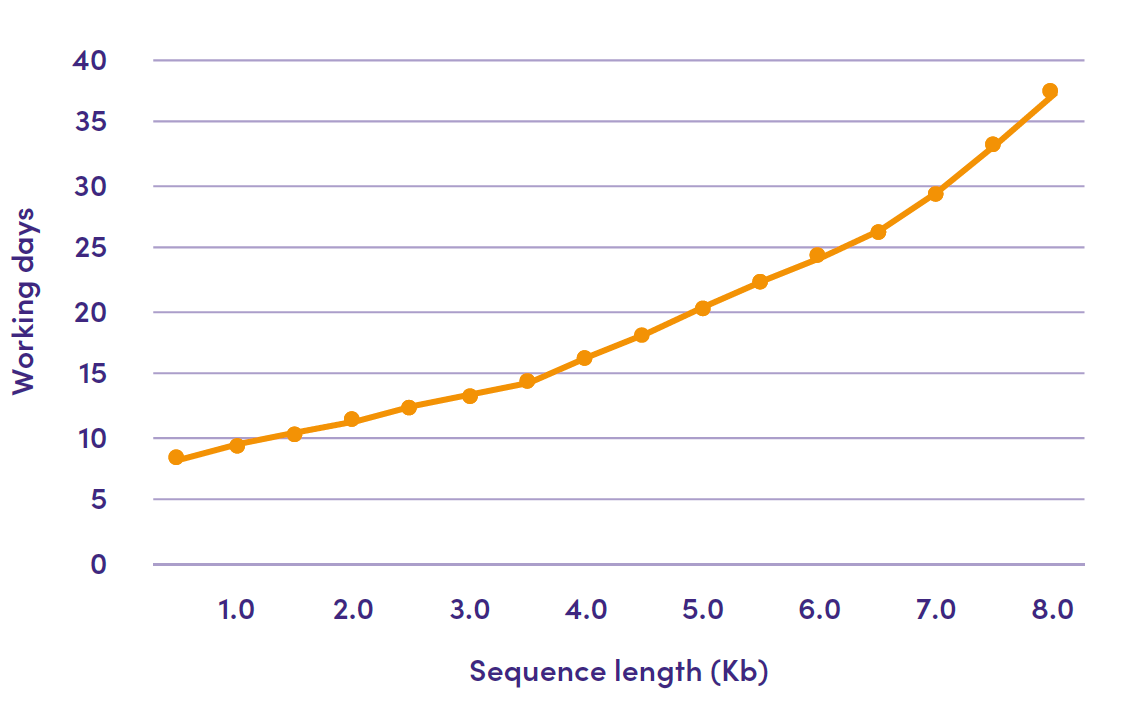
A second limitation with current technology comes from errors that are incorporated during DNA synthesis, resulting in significant post-synthesis steps being required to identify error-free sequences. Screening for error-free sequences involves cloning into a vector, transformation into bacteria, isolation of individual clones, and sequence validation by Sanger sequencing. As the length of the DNA sequence increases, the yield of error-free DNA decreases significantly such that with an elongation cycle efficiency of 99%, the theoretical yield calculation for an oligo composed of 1 kilobase is less than 0.01%.
Developments in DNA synthesis technology present opportunities to resolve these two bottlenecks and enable scale-up of mRNA vaccine development. Evonetix is developing technology that reinvents DNA synthesis with sophisticated gene synthesis capabilities in a benchtop device, putting control directly in the hands of scientists and allowing the synthesis of long, accurate DNA on demand.
Our patented Binary Assembly® process and thermal control via a unique DNA synthesis and assembly chip allow oligonucleotides to be automatically combined into double-stranded gene-length DNA and error-containing sequences removed, thus significantly improving the quality of DNA synthesized and reducing post-synthesis steps.
Outlook
mRNA vaccines have proven their value during the COVID-19 pandemic, and hold great promise for breakthroughs across a range of other indications, including personalized therapy. However, developing mRNA vaccines at scale requires access to gene-length DNA beyond what is possible today. Evonetix’s gene synthesis technology will be instrumental in enabling mRNA vaccines to achieve their potential.